Gokul Kannan (C3 Therapeutics)
The CEO of C3 Therapeutics tells us how they're building a new class of antivirals, discusses a useful mental model for understanding biology, how to manage risk, and exciting emerging science
Welcome to the 7th issue of Master Plan, a series of conversations with hard tech founders solving really difficult problems.
Gokul Kannan is the co-founder and CEO of C3 Therapeutics, a biotech company developing broad-spectrum antivirals. Gokul is one of the most passionate scientists I’ve ever met, and you can always learn something from his enthusiasm even if you don’t fully understand the details of his work.
Gokul introduces himself
I do science sometimes.
I'm currently a senior at UC Berkeley, but I have a very broad scientific experience. What that means is I’ve done projects with significant depth in biomaterials, drug delivery, bioinformatics, and medicinal chemistry, as well as astrophysics. I’ve been lucky to learn from mentors and collaborators in different fields.
So I've kind of got a very wide-ranging focus in my science, and all of that comes together in every project. We incorporate chemistry and physics ideas in biomaterials projects, as well as drug delivery projects. I’m fundamentally a scientist at heart.
I’m also pretty social and love to meet new people, so DM me on Twitter or send me an email (gokulkannan21@berkeley.edu) for more info/more in-depth discussion!
C3 Therapeutics - building a new class of antivirals
The idea behind our work in antivirals was originally spawned from us reading Dr. Todd Rider’s 2011 paper on DRACOs, but we realized there are details that still have to be developed for viability. DRACO is a protein-based therapeutic that selectively induces cell death in cells containing viral RNA. They wanted to use it as a prophylactic therapy, where you take the DRACOs and it’ll stop you from getting a sustained infection.
Chemically and biologically, there are a lot of challenges to overcome. However, the idea of killing an infected cell is dope.
And then we thought, can we restore the body's function to naturally kill infected cells? Because that's what your cells want to do. If they're infected, they self-destruct. But they can't because the virus stops it. So by restoring that function, we can nuke the virally infected cells and cure the virus in theory.
We found that concept exciting and got to thinking about those other approaches, initially based on a 1999 Nature Medicine paper from HHMI and WashU St. Louis, from Dr. Steven Dowdy’s group. They used modified caspase-3 proteins to kill HIV cells in culture, using a “Trojan Horse” strategy we’re trying to take advantage of.
Since 1999, science has gotten a lot better at drug delivery. We’re reframing what was previously a super hard delivery problem into a more tractable one, and also putting new spins on that ‘99 Nature Medicine method. That’s what really takes this cell-killing idea to the next level and makes it a transformative platform technology.
How would we go about this?
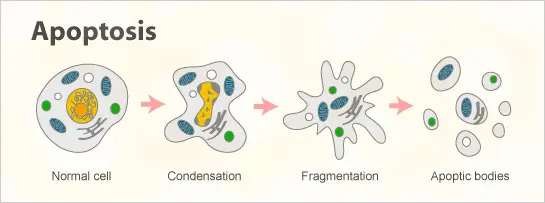
Well, because the virus blocks cell death, we need to start the cell death process at a very late stage. We’re targeting one of the last steps in the apoptotic process—so deep that it’s hard for the virus to reach it.
Here’s an awesome video of human cancer cells undergoing apoptosis.
And then by activating that process, we can kill the infected cells. This reorients the idea from a preventative one to a curative one, where we provide the machinery necessary for the cell to self-destruct.
The viruses need what are called proteases, which are processing proteins. These proteases basically act like scissors—they cut up other proteins and trim them to the right side to fit and help the virus propagate.
Caspase—which is the protein we're targeting—normally exists in what's called a procaspase form. This procaspase form is 2 symmetrical units connected by a linker peptide. That linker peptide gets caught and then the caspase activates.
By engineering that linker peptide to get caught by the viral protease (the scissors), the virus itself activates the cell death process. It’s pretty elegant—the virus is activating a booby trap to bring the whole house down and kill the cell and the viruses along with it.
Growing up with scientist parents
My parents co-founded Ashvattha Therapeutics. They’ve actually got a nanomedicine in clinical trials for the cytokine storm hyperinflammation (and potentially neuroinflammation) from COVID right now. They’re working on neuroinflammation associated with several neurological disorders.
It’s kind of exciting because my parents have always been outsiders to the biotech community, in some ways. They’re both immigrants and didn’t have it easy getting to where they were, so watching them go through an absurd amount of difficulty bringing their work to the clinic has been both an inspiration and a source of learning for me. They spent more than a decade building quietly from scratch, and it’s now starting to show up in a huge way.
I’ve gotten a front-row seat to what successful drug development looks like. In fact, they probably have one of the most pre-clinically robust data on their platform that anyone has ever had for a clinical-stage product. So watching them build out such a rigorous base of proof for their work has been awesome, and I think that carries over to all of my work and my desire to make sure that everything is safe for people and making sure I understand how stuff works.
The value of journal clubs
I run a journal club on the Gen Z Mafia server, which is a way to get a scope of who's into biotech and life sciences, especially as well as just generally deep tech. A journal club is like a book club, but we discuss scientific journal articles instead of books.
Knowing a specific niche subject area is very different from keeping up with the frontier of literature across a larger field.
You’ll often find, especially with Gen Z, people who know a lot about a very specific field. That’s not a bad thing, but what ends up happening is you’ll get a form of tunnel vision that can lead to oversights, especially in a field like life sciences, where you have very high-dimensional problems of the human body.
Therefore, it’s important to keep a broad overview of everything that’s going on, and that’s what we’re trying to get at with a journal club. We’re also targeting people with no experience with science at all who want to develop critical scientific thinking.
Astrochemistry and star formation
I recently put out a paper in astrochemistry with my mentor Dr. Richard Saykally and some friends.
We like to think that scientists have a very good picture of how the universe kind of came to be like, post-big bang.
But the truth is, scientists are still building a picture of the first few hundred million years, and one of the major gaps in our knowledge is how the very first stars cooled and formed.
If you remember the ideal gas law from high school physics, PV = nRT.
You've got this massive cloud of super-hot gas and dust. But stars are way denser than that and yet they’re formed from that cloud. So for this stuff to condense, it had to cool. As V decreases, so does T. The only way it can cool is by radiation, by releasing light. And each of those photons that get released, carries away with it some energy.
We think one way this could have happened is via a hydrogen-based mechanism. Where the H2 molecule helps cool the star by sending away light. But these stars had to cool to something on the order of 50 to 100 Kelvin at most. Otherwise, they would have formed a black hole instead. And we know that stars formed first.
That presents an issue because molecular hydrogen cooling sucks at low temperatures. It’s just terrible. But we’ve accepted it anyway because we can’t find a better explanation. We proposed this mechanism using a class of molecules called Rydberg molecules, including a molecule called H3—triatomic hydrogen.
At first glance, this makes no sense, but it (and a couple of similar molecules) form quite nicely in these types of primordial gas environments. We proposed that high-energy Rydberg states of H3 and other types of exotic, or previously thought exotic molecules, like helium hydride drove the cooling of the early stars instead of just H2.
If we're right, that means now we can align the timescale that we're pretty sure the stars formed with how those stars actually did. What this does help us understand is the missing piece in the cosmological puzzle of how the first stars really came to be.
The role of serendipity in science
When we wrote the astrochemistry paper, it was very serendipitous in terms of Dr. Saykally being my intro chem professor, and then also digging up some of his old data that nobody’s touched since 2015.
A lot of scientific breakthroughs are accidents or come from weird interactions. Even in biology, a lot of the field emerged by accident. We just need to be observant and think creatively, and the pieces will eventually start to fit together at very weird times.
Professor Jennifer Doudna was not looking for gene editing when she discovered CRISPR. In fact, she was originally recruited to Berkeley because of her work on structural biology. Completely different.
But she was very excited about bacteria. Having gone to a few of her talks, I think it’s amazing and inspiring how much she really loves this fundamental study. And from that, she was able to discover this repurposed gene-editing mechanism.
And obviously, there’s the canonical story that everybody tells us about penicillin, where it was a pure accident where the breakthrough came out of just observing that accident.
A large part of it is about being observant and creative, and not necessarily accepting the easiest explanation when things don’t really check out.
On preventing cancer migration
Dr. Sanjay Kumar is my faculty mentor (shout out to Joe and Kayla for being my amazing direct mentors), and his group was really where I grew into a very solid, rigorous researcher.
In the Kumar lab, I’ve really been able to develop a really good sense of rigor and ensure that everything I put out is as well-proven as possible. The research I’ve done there is related to understanding the mechanisms of cancer migration.
Glioblastoma is among the worst cancers. Pretty much everybody with it dies. And the reason for that is because of recurrence. You get glioblastoma, you get treated for it, and surprise: two years later, it’s back and worse. This is caused by what we call proneural to mesenchymal transition (the glioma analogue to epithelial-to-mesenchymal transition), or at least we think so now.
So your initial glioblastoma cells go into overdrive. They get super aggressive and run all over the place. And then they start to see new secondary tumors. These new secondary tumors are what cause death later on.
We’ve been developing hydrogels and micro-devices to understand how and why these glioblastoma cells move (beautifully described in Kayla’s recent review). Thanks to these, we’ve learned about not only the properties of migration itself but also “switches” that might be able to turn on and off that movement.
Our overall goal is to find the legs of these moving cells and figure out how to cut them off. If the cells can’t run away, the secondary tumors can’t form, and killing the first tumor will be enough. Our big step towards this is with hydrogel models and the subsequent understanding of how glioblastoma moves and acts.
On The Youth Project
I had very good mentorship and ended up hitting the right pieces of luck along the way to get excited about science. A large part of why I’m able to work on this antiviral stuff and start these projects is because I got excited early and stayed excited about science itself over time.
I co-founded The Youth Project in the US, and our overall mission is to ensure that young people around the world get the resources they need to make change around them. We focus on helping people make change in their localized areas, whether that’s through funding projects or running specific initiatives.
For example, I taught an engineering and robotics course at an orphanage in India, and the students from that course went on to participate in competitive events. And that was really exciting just to be able to watch them go from no engineering knowledge to being able to qualify and compete against highly-ranked schools.
The Youth Project has also built schools and sports clubs in India, helped homeless people find stable jobs and affordable housing across the US, and more. This is a way of extending my resources and helping people how I can, while also recognizing that I got really lucky.
The more people that are excited about science and get into science, the better the field gets. More creative people working on biology means we do better biology.
How to understand Biology
In some cases, we’re kind of stuck with the formality of biology and the dozens of names you need to memorize. In some subfields, it is what it is. But how I generally approach biology and chemistry—and how I would highly recommend everybody at least try this out—is to think from a physics perspective.
Instead of thinking from a pure biology perspective, approach the field like you would physics. If we look at Science on a scale, one of the most empirical sciences is biology and the least empirical science is math. Everything else exists on a spectrum.
The closer we get to the theory side and establishing things as low dimensional, understandable systems, the more we can predict and understand what happens.
For biology, a lot of the time, the only way to truly know something is to empirically prove it.
No one knew that adenosine kinase phosphorylates NAD until somebody literally observed adenosine kinase and found out that it phosphorylates NAD. But in math, you can just prove stuff based on other principles completely and entirely, and that’s rigorous and thorough.
It’s easy to say in biology that you don’t know something because we don’t have the data. But even if it’s wrong, I feel like it’s better to say we don’t have the data, and then explain how something is most likely going to work based on the physical principles and assumptions we made earlier.
If your assumptions are right, then you’re right. More often than not you’re wrong and you fail, but it is what it is.
With understanding and modeling biological systems as physical systems, part of it is figuring out what assumptions you can make and what details you can abstract away to understand it like a physical system.
The TLDR is to take these complex systems,
Make assumptions to simplify the parts that we care about
Build model systems based on physical principles to understand them
Validate the models with others or clinical data and iterate
Profit (and more importantly, improve human health)
How to learn Biology
There's a lot of spaces to get involved, and it really depends on what stage you want to.
For example, computer science has gotten more and more heavily integrated with biotech recently. Whether it’s on the molecular dynamics and simulation side, which actually is quite a bit of physics as well, or like -omics or big data and dealing with massive RNA or protein sequencing datasets.
There’s a place for everyone. It doesn’t matter what your existing expertise is. If you want to start at the ground level, it’s important to have a fundamental understanding of how biological and chemical processes work.
I would recommend building a niche of knowledge around one fundamental scientific subject. For example, if it’s chemistry, take some intro to chem classes, look through Khan Academy, and stuff on YouTube to get a good understanding of fundamental chemistry.
And then from there, you can start applying those same principles to understanding biological and physical systems. Every other science gets easier to learn once you know at least one fundamental science because you’ve got a framework or lens to view it through.
Or you could read a textbook if that’s your thing. I personally hate textbooks, but a favorite is Fundamentals of Momentum, Heat, and Mass Transfer by Welty, Wicks, and Wilson. I remember the whole thing because I like it so much.
On managing risk in biotech
There's always some inherent risk until you try a drug in humans. There’s a risk that either your animal model doesn't reflect what happens, your drug doesn't hit the thing you thought it would, it goes to the wrong spot, etc. There are a million things that could fail.
It’s a game of mitigating as much of that risk as possible. You also need to acknowledge that you could spend your entire life trying things outside the clinic and in animals all you want, but at some point, you just need to put the drug in a person.
We’re actually getting quite good at mitigating risk now. Not good enough, but getting better. We have things like organoid models, where we basically build out an organ on a chip—like a heart or a liver on a chip, and then test a drug on that chip and see how that metabolizes and acts on what is basically a tiny human liver.
This lets us study an extremely multifunctional liver that we would normally never be able to replicate. And it helps us dissect that and understand exactly what happens. So one example of mitigating risk is developing a deep mechanistic understanding of the lab.
Another way is by developing animal models. There are a ton of animal models for most things, and there are no animal models for many things. For example, Alzheimer's is very spicy. Everyone's excited about it, but our animal models aren’t actually representative. Therefore, most drugs that go into trials fail.
There are a few canonical hypotheses you’ll hear about Alzheimer’s, whether it’s tau tangles or amyloid beta deposits. Of the few major ones now, most of those have some aspects that are wrong because we’ve targeted them, hit them, and they failed.
If we had a good Alzheimer’s animal model, we would be a lot better off. But we don’t have a good animal model because animals don’t get Alzheimer’s.
It’s about finding the right models, both in-lab and in animals, to really mitigate the risk. But there’s always going to be some inherent risk, and there’s always a chance you’ll lose a couple hundred million on a massive trial that goes nowhere.
VC metrics for biotech
Investors are generally looking for 2 things:
How much have you de-risked this?
What is the market potential, either through therapeutic development and a clinical trial success or (more likely) an exit by big pharma?
Big Pharma acquires a lot, and they acquire big. You’ll see a startup get excited about being acquired for $100M. But in biotech, it’s like damn, only $100M? Gilead has acquired 4-5 companies in 2020—the last one was $21 billion. The one before was like $5 billion. And these aren’t exceptions. Exits are really the go-to and it’s important to keep in mind someone you can sell to.
In terms of early-stage fundraising, every company is different. People might be asking more questions about the core science, like:
Do you understand the delivery of your drug? Where does it need to go? How does it act in each area? Can you get it where it needs to go initially?
How potent is it? If it’s not potent enough, you’ll see no effect unless you give too much of it.
How much have you de-risked this in animal models?
The earlier stage you are, the vaguer it gets. But right now, there’s no way to have true product-market fit in the same way you would look for it in a tech company.
It's just science, which I like a lot.
Building a Team
In terms of building the core scientific team, I tend to be most concerned about finding people who approach problems in the right ways and have the right expertise to complement your own.
Our team is currently 3 people: Me, Praneeth, and JZ.
I bring fundamental drug development and drug delivery knowledge, along with very strong experience in biomaterials and tissue engineering, chemistry, and bioinformatics
Praneeth brings a super-extensive knowledge of animal models and really solid cell/molecular biology experience. This guy does like 90 mouse experiments on the reg, it’s just casual for him.
JZ helps us translate this and get it out there in terms of raising money, getting in touch with the right people, and helping us build out everywhere except the science. He’s absolutely crushed it leading Matrix.
Praneeth and I really gel well on the science because he also approaches these problems from a physics and engineering perspective, as opposed to just a biology perspective. I’m not too afraid of making a couple of assumptions to simplify a complex landscape, and neither is Praneeth.
I would encourage everyone building a team to find people who approach the problem in the same way you’d like to while filling gaps in your experience that you can learn from.
How to communicate highly-technical ideas
What's important is to understand the high-level impact and a general conceptual understanding of how it works—drop the names. Biology can be so semantic, and there’s so much you’ve got to know to get a thorough understanding of something. But you don’t need an understanding of every single nitty-gritty bit to learn and apply different ideas.
It’s important to convey conceptual and mechanistic understanding. For example, in Moderna’s mRNA vaccines, the mRNA carries a message to produce this protein, and then your immune cells will recognize and develop immunity against that protein after it gets produced.
This is a very high-level conceptual understanding of the mRNA vaccine process, and it’s accurate enough to build other ideas on top of. For example, you’re able to think: if we can send a message with mRNA for vaccines, maybe we can send messages with mRNA for other things. Like antivirals. Surprise, that’s what we’re doing.
We’re planning on taking this core mRNA idea, a concept very beautifully proven by Moderna, into a new space with quite a bit of exciting new science we’ve applied to it.
PROTACs—a very exciting emerging field
The most exciting emerging field, to me, is PROTACs. These are awesome. They’re proteolysis targeting chimeras.
You might’ve heard of thalidomide, which was a drug in the 50s and 60s that caused birth defects in babies when it was given to pregnant women for morning sickness. Later, people found out that that thalidomide is something we call a molecular glue.
A molecular glue takes a degrader protein and another protein and sticks them together.
In thalidomide, it took the protein necessary for limb formation and tied it together with a degrader protein. So the degrader protein degraded the limb formation protein, and babies were born without limbs.
Now, that’s kind of a spicy mechanism. We don’t want to destroy limb formation, but we can use it for other things.
Here’s the really solid benefit:
Normally, for each protein you want to target, you need a small molecule to inhibit it. A small-molecule inhibitor, normally a drug, just sticks to protein and blocks it up until the protein dies.
With a PROTAC, you actually cause protein death. So you drag the degrading protein over your protein of interest, and boom. Gone. Drag it over to the next one, and it’s gone. So you keep destroying these proteins, and you can get a high potency on top of that.
There’s this idea you can target proteins you can’t normally hit other ways. There’s a variety of proteins we call undruggable. And PROTACs actually offer us the potential to drug those undruggable proteins.
It’s still really emerging in the clinical landscape. It’s very much a medicinal chemistry thing—I haven’t seen much of it outside of the medicinal chemistry and biopharma community. But there’s definitely an undertone of excitement about these things.
Bioprinting and organoids—also very exciting
Developing high-density, 3D-printed tissue is an emerging and kinda underrated area of research. The goal is to 3D print dense tissue nicely. The real end goal of most bioprinting is to develop transplantable, off-the-shelf organs, where if someone needs a heart or a liver you can just print it out and give it to the patient right now.
The problem is that we can’t print up to requisite densities. And we especially can’t do so with any nicely vascularized tissue so that nutrients can reach everywhere. There are a number of labs working on those vascularization and density problems.
The development of organoids is also extremely cool. Not only for the problem of off-the-shelf organs, but it’s also heavily applied to drug testing or applications where you can start to build out an organ in a dish.
And one of the really beautiful things about this is in some cases, you can simply give nature the initial seed and let it go. If you seed an organoid just the right way, it can actually progress and form the full organoid itself without too much intervention. We can then study biology in those organoids and relate that to human biology beautifully.
One of my favorite scientists—and my mentor this summer (paper coming out soon!)— is Dr. Takanori Takebe, at Cincinnati Children’s Hospital and Tokyo Medical and Dental University. He’s an actual god when it comes to this and does truly transformative work.
Gokul’s Master Plan
Tying it back to physical principles, I believe that the vast majority of biological problems can be simplified using the right assumptions and the right models into physically understandable systems.
I want to find those systems, model them, and then build therapeutics to treat those diseases.
In the case of viral disease, our company is basically able to simplify complex viral disease into a very straightforward, simple thesis. I think this methodology can be applied across many fields using what we call platform technologies.
With our company, we don’t just want to cure one viral disease. The concept we’re working with applies to the vast majority of other viral diseases—we can hit everything that has a protease.
So building these platform technologies lets us solve a ton of problems at the same time.
I want to build platform technologies to treat entire classes of diseases. That’s the master plan.
Thanks for reading! You can follow Gokul on Twitter @gokoolerthanyou if you haven’t already. And if you’ve learned something from this newsletter, try sharing it with your friends.
If you’d like to read more about building ambitious companies, sign up here. You’ll learn how founders go from a crazy idea, to hiring a team, and solid execution.
For any questions, feedback, or requests, send them to me via Twitter.